Because the arguments advanced in Explore Evolution require readers to have a level of knowledge beyond that offered in the book or in standard high school or college introductory biology texts, this primer on developmental biology and evolutionary developmental biology may be useful for some readers.
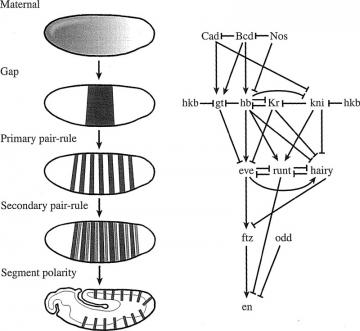
Sean B. Carroll, Jennifer K. Grenier, and Scott D. Weatherbee (2001) From DNA to Diversity: Molecular Genetics and the Evolution of Animal Design, Blackwell Publishing:Cambridge, MA, p. 59, fig. 3.5
A good resource for the basic background necessary is From DNA to Diversity by Sean Carroll, or his book Endless Forms Most Beautiful. Carroll describes developmental biology in terms of "tool kits" of genes. A group of genes which interact and regulate one another's expression would form such a tool kit, and such kits can operate as somewhat independent modules. "Modularity," explains Rasmus Winther, "is central to the current evolutionary developmental biology synthesis" (R. G. Winther, 2001, "Varieties of Modules: Kinds, Levels, Origins, and Behaviors," J. Exper. Zool. (Mol. Dev. Evol.) 291:116 129). How those modules interact is controlled by other toolkits, producing a hierarchy of kits. For example, in Drosophila, five hierarchal tiers of regulation (maternal effect, gap, pair-rule, segment polarity, and homeotic) are involved in organizing the body pattern along the axis from head to tail of the developing embryo. Each segment of the body is produced through interactions between gene products translated from mRNAs deposited in the egg by the mother, transcriptional activation of genes in the egg by such maternal activators, and combinatorial action of segmentation gene products (tool kits) to refine the expression patterns of many zygotic genes.
Explore Evolution skips any presentation of this regulatory network of tool kits and invites the students to compare the regulation of body segmentation in fruit flies and wasps, declaring: "The body segments of some wasps arise from developmental pathways that are entirely different from those of fruit flies, and even from other wasps" (EE, p. 44). The student has no way to evaluate this claim, nor to judge its significance, because high school biology texts rarely cover developmental biology, and EE certainly doesn't offer that background. In the diagram of Drosophila segmentation shown above, the seven stripes that make up the expression patterns of the primary pair-rule genes hairy and even-skipped are controlled independently. That is, different regulatory elements control the expression of different stripes.
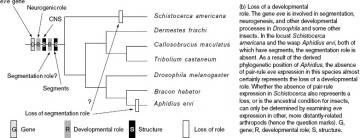
Image from Gregory A Wray and Ehab Abouheif (1998) "When is homology not homology?" Current Opinion in Genetics and Development. 8(6):675-680
In the wasp species mentioned in Explore Evolution, the same segments are produced even though the even-skipped gene is not expressed at the point when it would be in Drosophila. Nevertheless, the gene which normally follows the expression of even-skipped (engrailed) is not affected, and instead, acts to facilitate normal patterning and segmentation. The wasp species lays its eggs in other insects, and the egg develops as a parasite within the other insect. In order to survive in this environment, the egg structure is modified in many ways, and expression of even-skipped during early development is apparently affected by these changes. The gene still exists, and is expressed at other times. Because of the modular nature of developmental toolkits, the eve toolkit can be switched off early on without affecting later stages in development.
Examining the details of this evolutionary process led researchers to make specific predictions. Observing that the eggs of other parasitic wasps have similar adaptations, and noting the similarities of later developmental patterns across insect species:
we would predict that changes in patterning mechanisms will occur in other insect taxa that exhibit shifts in life history that favor the loss of yolk or early cellularization. By contrast, insects with ectoparasitic or free-living life histories will exhibit patterning mechanisms that resemble those of Drosophila.Miodrag Grbi and Michael R. Strand (1998) "Shifts in the life history of parasitic wasps correlate with pronounced alterations in early development," Proceedings of the National Academy of Sciences. 95(3):1097-1101
Not only does an understanding of the full complexity of developmental patterning clarify the evolutionary basis for this variation in developmental pathways, it yields new, testable hypotheses. The process of scientific inquiry rests on that cycle of proposing hypotheses, making predictions, and testing those predictions. An inquiry-based textbook would revel in those opportunities, would show students how scientists construct new hypotheses and test them, and would encourage students to make and test their own hypotheses. Explore Evolution is not inquiry-based. Their discussion of subjects of active research consistently gives the impression that unanswered questions must be unanswerable. This attitude is not merely unscientific, it is anti-scientific.
Sean B. Carroll, Jennifer K. Grenier, and Scott D. Weatherbee (2001) From DNA to Diversity: Molecular Genetics and the Evolution of Animal Design, Blackwell Publishing:Cambridge, MA, pg. 5.16, 144
Observations of the way that genetic changes can affect development of homologous structures have led scientists to make many fundamental and testable hypotheses about the evolution of the shape of the body. Some developmental biologists argue that the independent control of distinct regulatory tool kits is one of the most important concepts in the spatial regulation of gene expression in animal development, and plays a central role in the evolution of morphological novelty. Certain tool kits may be more modular than others, which could explain the pattern Wagner (2007) observes, that developmental variation in homologous characters is not randomly distributed, but affects some aspects of development more than others.
On the topic of the evolution and diversification of homologous body parts, Carroll offers two excellent examples, the insect hindwing and the vertebrate forelimb (figure at right). He explains how homologous structures, such as the fly haltere and butterfly hindwing are determined by different genes. There is conservation of selector gene expressions, in both cases the insect hindwing is ultimately controlled by Ubx. The same regulator gene acts on different sets of target genes to create variation in morphology in these lineages. Development of the vertebrate forelimb is much the same, with the same selector gene Tbx5 controlling different target genes to produce different morphologies in birds and humans, which are homologous in their basic structure but show variation in the developmental pathways.
The inadequacies in Explore Evolution are especially apparent in its treatment of gut development. They fail to cite the source for their claims about the different origins of the vertebrate gut (though it is presumably Gavin de Beer, 1971, Homology: an Unsolved Problem. Oxford: Oxford University Press; 1971). Because they are citing a passing reference in a 37 year old summary, their terminology is imprecise, failing to clarify what cells they are talking about, or to provide any background information that students might need to understand what the embryonic cavity might be, or how the gut develops from it. Students have no hope of understanding the example. This would be surprising in any other textbook, but confusion seems to be an objective of Explore Evolution.
[The paragraphs in italics may be too speculative, and might be worth cutting. I'm leaving them in so that I remember what I figured out about this, but won't weep if they're deleted.]
[The cavity they refer to is formed early in embryonic development. When the embryo has only a few hundred cells, it forms a hollow sphere with walls one cell thick. One wall pushes in, leaving two walls (the outer cells are called the ectoderm, the inner are endoderm) with a gap between them, and a central cavity (called the yolk sac). The gap between the walls fills with a third type of cells, called mesoderm. The gut forms when a tube of endoderm closes off from the rest of the yolk sac. Different groups of vertebrates form that tube in different locations within the yolk sac. This does not mean that homologous cells are not involved, nor that homologous genes are not involved. Because the endoderm forms when the outer wall pushes in, a small shift in the location of the beginning of that endoderm could mean that descendants of homologous cells (cells which originated from similar patterns of cell division, or which express the same toolkit genes because of a shared developmental trajectory early on) would wind up in very different parts of the embryo.]
[Another possibility is this simply illustrates the modularity of the developmental tool kits that control gut development. Because the genetic tool kit controlling gut development has not been fully worked out, it is not known what signal initiates the formation of that tube, nor how that signal differs between different lineages of vertebrates.]
[These and other hypotheses about the formation of the gut are subjects of ongoing scientific inquiry.] The development of the vertebrate gut has not been as intensively studied as other structures, and the process by which scientists gain new knowledge and test new hypotheses can be easily presented to students with some background in developmental biology. As Didier Y. R. Stainier observes:
[The gut's] location deep within the body has until recently hampered investigation into its formation. The patterning of the gut is one of the fascinating issues that pertain to the development, function, and homeostasis of this understudied organ.
At first glance, the gut looks deceptively simple . Yet in evolutionary terms, the gut, as an endodermal organ, predates any mesodermal organ, and it has reached a level of complexity and sophistication that is only starting to be appreciated.
Over the past decade or so, studies in a number of invertebrate and vertebrate model systems have provided insights into the genes and cellular mechanisms regulating endoderm formation. These studies have revealed a high degree of conservation in some of the transcriptional regulators of endoderm formation; for example, members of the Gata and Forkhead transcription factor families have been implicated in this process across the phyla, although the intercellular events regulating endoderm formation appear to be more divergent. The Wnt signaling pathway has been implicated in the formation of the endoderm in [invertebrates], whereas transforming growth factor b (more specifically Nodal) signaling has been implicated in the formation of the endoderm in vertebrate embryos. However, this apparent lack of conservation of the signaling pathways regulating endoderm formation probably reflects our incomplete understanding of the process. Indeed, we have not yet gained sufficient knowledge to control the efficient differentiation of mammalian stem cells into endoderm, meaning that the investigation of endoderm formation must proceed using multiple approaches in multiple model systems.
Compared to readily accessible organs such as the limb, or to organs such as the heart and pancreas that are the focus of resourceful charities, the gut has been left behind. However, it is clear from the few vignettes presented here that the many fascinating developmental, evolutionary, and medical aspects of the gut will continue to attract much attention and generate pertinent information.Didier Y. R. Stainier (2005) "No Organ Left Behind: Tales of Gut Development and Evolution" Science 307(5717):1902-1904
New technologies and improved techniques are only beginning to allow us to investigate the forces driving the development of the vertebrate gut. Scientists know that situations like this are thrilling chances to dramatically increase our understanding of the world. The aversion to inquiry that runs throughout Explore Evolution could not be clearer than in its brief, dismissive and submissive handling of this area of active research.
Fortunately, scientists can refer to the development of other structures to help inform this research. Mary Jane West-Eberhard describes one example:
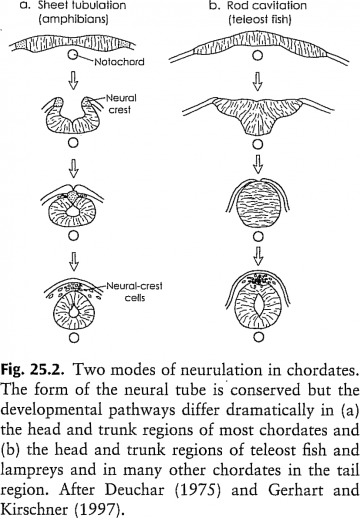
Image from p. 495 of Mary Jane West-Eberhard (2003) Developmental Plasticity and Evolution, Oxford University Press:Oxford. 794 p.
Perhaps the most impressive illustration of endpoint conservation despite different developmental pathways occurs in the ontogeny of the chordate neural tube. The neural tube is a distinctive embryonic trait at the phylum level a "phylotypic" or "archetypical" trait. Not only is it present in all chordates, but it is essential to normal development of the nervous system and other structures. In most chordates, the neural tube of the head and trunk is formed when an epithelial sheet, the pre-ectoderm, rolls inward to form a tube, whereas the neural tube of the tail forms by coalescence of cells as a solid rod that then becomes hollow to form a tube (figure [at right]). In teleost fish and lampreys the latter mode of neurulation prevails over the entire body length. Clearly the chordate neural tube can be formed by quite different morphogenetic means, and the exact path and means of morphogenesis are not linked closely to the developmental fate of cells.
Because of highly flexible developmental interactions that include such devices as induction by an organizer of multipotent cells, and highly flexible cell migration, this major difference in developmental origin of the neural tube does not affect the ability of the embryo to organize itself into the standard chordate body plan. The neural plate always arises near the notochord, and the notochord is always surrounded by the neural tube, somites and gut. This arrangement in turn accommodates numerous specializations of later development. Even though neural tube formation and other processes may undergo circuitous evolutionary change, the chordate body plan is conserved.
Given the developmental divergence, should we regard the neural tubes as homologous in all chordates? On one level, yes: the phylotypic stage is conserved by flexible mechanisms held in common due to common descent. On another level, no, because developmental sequence may reveal convergent derivations of such structures as the neural tube. Examples like this support the conclusion that "the similarity of homologous characters cannot be explained or caused by the invariance of developmental pathways" (Wagner, 1989, p. 1163), even though developmental pathways may illuminate homology.Mary Jane West-Eberhard (2003) Developmental Plasticity and Evolution. Oxford University Press:Oxford. 794 p., pp. 495-496. Citations omitted, except for Wagner, G. P. (1989) "The origin of morphological characters and the biological basis of homology." Evolution 43:1157-1171.
The modularity of developmental toolkits allows these changes in the timing or location of development. Redundancies in the developmental pathway allows the removal of certain stages in development without preventing development of the final form, and the self-sufficiency of tool kits allows the same structure to originate at a different stage in development or from a different part of the organism. This does not undermine the assessment of homology, it merely shows that the definition of homology offered in Explore Evolution is inadequate, an error which, once again, undermines their treatment of an important topic.