INTRODUCTION
When we try to explain evolution to those who do not know much about it, one of the problems we have is the definition of what counts as evolution. In part, this is because some of the definitions found in the scientific literature, including textbooks and popularizations of evolutionary theory, use technical terms that do not seem to convey to the public that evolution explains the diversity of living forms. In part, it is also because the popular idea of evolution as it is found in dictionaries, science fiction, and philosophical potboilers is a holdover of concepts that have long been abandoned in the biological sciences, if not in theology or in the "science" of popular media.It is important to understand these various definitions relative to each other and to show that accepting evolution as defined in the sciences does not commit one to accepting another form, as proposed by theology or philosophy. In particular it is important to realize that, contrary to many nonscientific uses of the term, evolution is neither a progressive process — modern theory does not make it inevitable that the latest is the best — nor can we expect to be able to predict the "next step" in evolution.
To understand the current meaning of the word "evolution", it is useful to take a journey through history and trace the various meanings of the word "evolution" as the modern view takes form.
THE HISTORY OF "EVOLUTION"
Evolution before Darwin
Initially, the Latin word evolutio meant the unrolling of a scroll. This gives the flavor of the inevitable recitation of a story or message. The story is already written, and all that is required is to "read off" the message in an orderly fashion from beginning to end. "Evolution" was therefore a natural term for the early developmental biologists, such as the Dutch entomologist Jan Schwammerdam in 1669 and Charles Bonnet in the 1740s, to apply to the development of an embryo (Richards 1992a, 1992b; Mayr 1982). This appears to be the first recorded use of the term in a biological context.Early evolutionists, like Jean Baptiste de Lamarck and Erasmus Darwin (Charles's grandfather), believed that species also changed in such a predetermined fashion (by ascending the Ladder of Nature, called the scala naturae). The term "evolution" was applied to the transmutation of one species to another in the early years of the 19th century, by Lamarck's disciple Etienne Geoffroy Saint-Hilaire and Geoffroy's student, embryologist Etienne Serres, to describe such views. Charles Lyell, in the influential second volume of his Principles of Geology (1833), which Darwin received during the voyage of the Beagle and which prepared him to formulate an evolutionary view, attacked Lamarck and Geoffroy on transmutation, and borrowed the term "evolution" from Serres to describe their views. By 1833, then, when Lyell's work was published, it had become common usage to equate "evolution" with the transmutation of species.
So evolution originally referred to the supposed series of changes that a species was predetermined to undergo, in the same way that an embryo is preprogrammed to develop. Such views persisted into the first half of the 20th century, despite Darwin's theory, which asserts no such predetermined series or stages. These views are called orthogenetic ("straight line") theories of evolution (Mayr 1982) and are in great disfavor today. All that persists of that original meaning is that species change over time. However, the use of "evolution" in its original sense is still common in astronomy to describe the sequence of stellar development (Gould 2000).
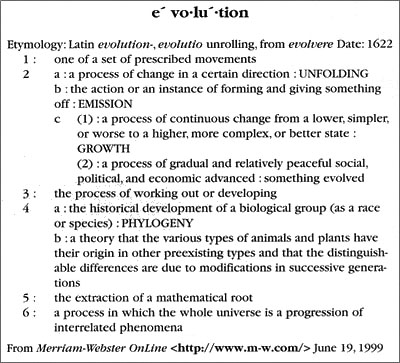
Darwin's view of evolution
Before Darwin, transmutation of species generally meant that a species as a whole changed into a more complex species through some (unspecified) process. Darwin intended no such meaning when he introduced evolutionary theory in On the Origin of Species (1859). First, he realized that change was not necessarily a process of increasing complexity or perfection. Second, he proposed a specific mechanism that explained why new species were different in appearance and behavior — natural selection. Third, and most important, Darwin saw that the origin of a new species did not involve an entire species' undergoing change. He saw that the origination of a new species might occur in only a part of the parental species — in a subpopulation. The remaining populations of the original species could remain unchanged.Species to Darwin were just permanent varieties. The species he had studied in the wild, and those he read about in the work of others, and most of all his alternately loved and hated barnacles (he spent 9 years cataloguing modern and fossil barnacles before publishing the Origin), all exhibited variation, including what were then called "races", or subspecies. This is the meaning of "races" in the subtitle of the Origin ("The preservation of favoured races in the struggle for life") — varieties within a species.
The fact that speciation occurred in subpopulations implied that species shared common ancestry with others that had split off from the same or related parental stock. So Darwin concluded that there was an "insensible series" of varieties — or what we would speak of today as genetic variants — from geographic differences to fully distinct but interfertile varieties or races, to distinct and mutually infertile species. This conclusion is not generally accepted today. The contemporary view is that species arise not from genetically distinct races, but from local and isolated populations that may initially be much the same genetically as the main populations of a species.
However, Darwin did not use the word "evolution" in the first edition of the Origin (although he used "evolving" in the sense of "unfolding" in the final paragraph). Only later, in the Descent of Man (1871; see Gould 2000), was Darwin forced to adopt the term "evolution", partly because it was in common use, and also because his associate Herbert Spencer had used the term two years before Darwin went public on evolution. The fact that the ideas he outlined are often included in the general understanding of "evolution" causes some confusion, which can be alleviated by carefully distinguishing among the components of Darwin's theory.
The main components of Darwin's theory are
- that species change (transmutation: Darwin's preferred term was "descent with modification");
- that related species are descended from a common ancestor (common descent);
- that the main mechanism by which species become distinct from one another is natural selection; and
- that species arise geographically near to their ancestor (biogeography).
In the book that effectively crystallized the Modern Synthesis of genetics and Darwinism, Ronald Fisher began by saying
Natural Selection is not Evolution. Yet, ever since the two words have been in common use, the theory of Natural Selection has been employed as a convenient abbreviation for the theory of Evolution by means of Natural Selection, put forward by Darwin and Wallace (Fisher 1930: xi).Fisher may be historically inexact, but there is a solid point here: natural selection is what many people mean by "evolution". This impression is reinforced by the writings of such luminaries as Richard Dawkins, who makes selection the core of his view of evolution.
But natural selection is also the mechanism by which species do not change. Selection only forces change if a population is not well-suited to competing for resources or overcoming risks in its local environment. If a species is well-adapted, then selection will inhibit change; this is called balancing selection. Thus, we have to distinguish between the mechanism that is sometimes an agent for change from the process of change itself (see Figure 1).
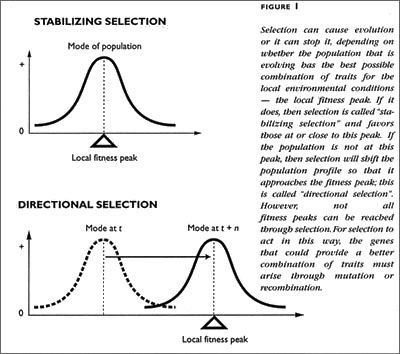
Before Darwin, the prevailing view was that species are types. A type was both a means of identifying and classifying an organism and also a force that caused it to exhibit certain physical characteristics. The type made that organism what it was. Organisms that varied too far from the type were "monsters", degradations of the type (for example, a French biologist contemporary of Linnaeus, Georges Buffon, thought that evolution was a degradation of a number of created types; Lovejoy 1959). Entire species were called monsters, because they were too far removed from the central type of a family or genus of organisms. Such central types were called the archetype by Richard Owen.
Darwin imagined the archetype as an actual historical ancestor — that the variation present in an ancestral population could be the basis for descendant populations both to share important identifying characteristics and to differ significantly in some ways from the ancestor. Furthermore, he saw that neither the archetype nor the type of a single species exerted any influence on the subsequent history of a lineage. For Darwin, types were just the most common form of a species or genus. The type might remain stable, or it might change. In modern terms, we would say that the type is just the mode of the distribution of species' characters. What causes the type to remain stable or to change is another matter. Whether the type changes or remains stable, the cause might be selection or some other process, such as random drift.
Evolution after the discovery of the gene
Modern definitions of evolution are based on the fact that all organisms living in breeding populations are generally quite similar, but no two individuals are exactly alike. They have differences due to their heredity, their upbringing, and their life histories. Some of these differences are of evolutionary significance: all of them involve their genes in some way; their dispositions to grow in particular ways, their ability to react to and make use of their environments, and even their abilities to deal with disease and injuries are genetically influenced, but not necessarily determined. Selection acts at base on genetic differences among organisms, not on an individual gene. Moreover, these differences have to occur in populations by virtue of the normal processes of genetic recombination in reproduction.Darwin's theory was extended by the so-called "biometrics" movement (which is the foundation of both statistics and population genetics) and melded together with Mendelian genetics beginning about 1920. This culminated in the Modern Synthesis (henceforth, the Synthesis) forged in the works of Fisher, Theodosius Dobzhansky, Ernst Mayr, JBS Haldane, Sewall Wright, and Julian Huxley between 1930 and 1942. For the architects of the Synthesis, it was natural to use the powerful set of theoretical and analytic techniques of genetics to define evolution (see references and discussion in Bowler 1984). Thus, Huxley, summarizing the views developed by Dobzhansky and others in the development of the Synthesis wrote:
Mendelism is now seen as an essential part of the theory of evolution. Mendelian analysis does not merely explain the distributive hereditary mechanism: it also, together with selection, explains the progressive mechanism of evolution (1942: 26)and
[W]hat evolves is the gene-complex; and it can do so in a series of small if irregular steps, so finely graded as to constitute a continuous ramp (1942: 68).The "allele-frequency" definition of evolution has survived to become the "standard" definition in textbooks and discussions about the nature of evolution. Here is a more-or-less random collection of quotations from various sources to illustrate how different views have developed based on this initial insight.
Biological evolution ... is change in the properties of populations of organisms that transcend the lifetime of a single individual. The ontogeny of an individual is not considered evolution; individual organisms do not evolve. The changes in populations that are considered evolutionary are those that are inheritable via the genetic material from one generation to the next. Biological evolution may be slight or substantial; it embraces everything from slight changes in the proportion of different alleles within a population (such as those determining blood types) to the successive alterations that led from the earliest proto-organism to snails, bees, giraffes, and dandelions (Futuyma 1986: 7).
[E]volution can be precisely defined as any change in the frequency of alleles within a gene pool from one generation to the next (Curtis and Barnes 1989: 974).
The fundamental evolutionary event is a change in the frequency of genes and chromosome configurations in a population (Wilson 1992: 75).
On the simplest perspective of all, biological evolution is analyzed initially as changes in allelic frequencies at a single locus. More complicated phenomena must be explained by means of combinations of these minimal units (Hull 1992: 185).
Natural selection deals with frequency changes brought about by differences in ecology among heritable phenotypes; evolution includes this as well as random effects and the origin of these variants (Endler 1992: 221).
Since evolution may be defined as cumulative change in the genetic makeup of a population resulting in increased adaptation to the environment, the fundamental process in evolution is change in allele frequency (Hartl 1988: 69).
Organic ... evolution, or biological evolution, is a change over time of the proportions of individual organisms differing genetically in one or more traits; such changes transpire by the origin and subsequent alteration of the frequencies of alleles or genotypes from generation to generation within populations, by the alterations of the proportions of genetically differentiated populations of a species, or by changes in the numbers of species with different characteristics, thereby altering the frequency of one or more traits within a higher taxon (Futuyma 1986: 551).Notice that some say that observable change in the frequencies of alleles is sufficient to define evolution, while others, such as Futuyma, think it necessary to go into more detail. For the purpose at hand, the dispute is unimportant.
A more important controversy, however, is between the proponents of the allele-frequency definition and those who reject it altogether as too narrow:
I pointed out more than a decade ago (1977) that the reductionist explanation, so widely adopted in recent decades — evolution is a change in gene frequencies in populations — is not only not explanatory, but is in fact misleading. Far more revealing is the definition: "Evolution is change in the adaptation and in the diversity of populations of organisms" (Mayr 1988: 162).
Evolution may be defined as any net directional change or any cumulative change in the characteristics of organisms or populations over many generations — in other words, descent with modification... It explicitly includes the origin as well as the spread of alleles, variants, trait values, or character states. Evolution may occur as a result of natural selection, genetic drift, or both; the minimum requirements are those for either process. Natural selection does not necessarily give rise to evolution, and the same is true for genetic drift (Endler 1986: 5).
Population geneticists use a different definition of evolution: a change in allele frequencies among generations. This meaning is quite different from the original; it now includes random as well as directional changes ..., but it does not require the origin of new forms. It is roughly equivalent to microevolution (subspecific evolution; macroevolution involves major trends, or trans-specific evolution...). Unfortunately, the use of the population genetics definition often results in an overemphasis on changes in allele frequencies and an underemphasis on (or no consideration of) the origin of the different alleles and their properties. Both are important in evolution.... An additional problem is that, for quantitative genetic traits, the frequencies of alleles at many contributing loci can change while the overall mean and variance of the trait remain roughly constant (Endler 1986: 7-8).And even those who stress the genetic character of evolution sometimes take a broader view:
Evolution is a directional and essentially irreversible process occurring in time, which in its course gives rise to an increase of variety and an increasingly high level of organization in its products (Julian Huxley cited in Newman 1956: 278).These examples illustrate that there is a wide range of approaches to defining evolution and that "experts" disagree over what to emphasize in their definitions. Some think that genes are a very good place to start, while others insist that important concepts about evolution are not captured in allele-frequency definitions. However, when it comes down to the nature of the evolutionary process, much of this is a matter of semantics — what to spell out and what to leave implicit. Despite the superficial differences in these descriptions, the apparent disagreements do not usually entail differences of opinion about what happened in the course of evolution, at least not in broad outline.
ISSUES IN EVOLUTION
Macroevolution
Creationists, and anti-evolutionists in general, frequently claim that macroevolution is not deducible from the allele-frequency definition of evolution. They very often claim that there are barriers to changing beyond the "kind" (an ill-defined term with no fixed meaning, which seems roughly equivalent to "species") — although such change has been observed many times as new species have been observed to evolve from old ones. This is the lowest level of macroevolution, as we will see in a moment.Some anti-evolutionists even allow for evolution of one species into another, but deny that the emergence of "major" groups, such as families or orders in the Linnaean hierarchy, can be the result of microevolutionary change. Often, this concession to microevolution is made only to accommodate the species diversity we see today from the necessarily restricted variation among the original "kinds" that are supposed to serve as the founding populations at the Creation or that were carried on the Ark.
Even among scientists, the term "macroevolution" is a vague concept. Many authors think that there is a qualitative difference between adaptive evolution and the origins of higher taxa or forms. In the original formulation, Y'uri'i Filipchenko (in 1927) used the term to mean origination of a novel species by splitting from an ancestral species — what we now call speciation or cladogenesis. Today it is more widely used to mean "large-scale" change, such as the evolution of novel "body-plans", "grades" of ecological niche specialization, or "key innovations".
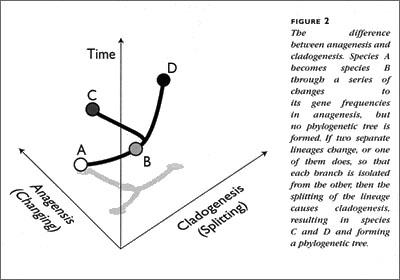
Those who prefer the allele-frequency definition of evolution argue that every such novelty began as minor variations on a theme in the origination of a slightly different species and that large- scale changes are the result of continued evolution of this kind over large periods of time. Often they think that evolution is always gradual (anagenetic) and that evolutionary trees (phylogenies) are just the additive sum of these gradual changes. Nobody denies either cladogenesis or anagenesis these days, but there is a fair bit of debate over the right mix (see Figure 2).
Varieties of evolution
Let us now look at the surviving meanings of evolution in order of increasing exactness, along with the names of some of the scientists with whom the ideas are associated (Bowler 1983, 1984; Mayr 1982; Mayr and Provine 1980; Ruse 1979/1999; see Figure 3).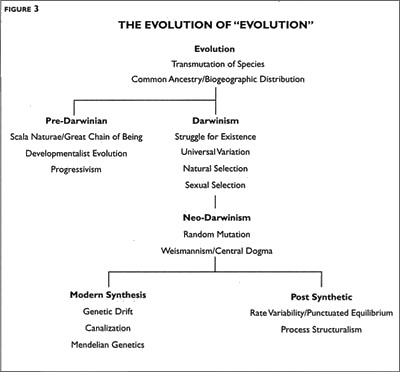
I take the broadest definition of biological evolution to be:
- Transmutation (descent with modification): This is the notion that new species emerge from existing species and that all existing species are the product of change in older ones. Early transmutationists: Lamarck, Erasmus Darwin (Charles's grandfather), Saint-Hilaire, Robert Chambers (author of the Vestiges of Creation, first published in 1844), and Charles Darwin. This view was common by the 1830s, and Darwin did not invent the idea.
- Common ancestry: Related species have changed from a common ancestor species; that is, the reason that species are similar and are related in classification is because they have evolved from a shared ancestral species. This is also called phylogenetic change, or more simply, phylogeny. In a limited way, both Lamarck and Erasmus Darwin proposed common ancestry, but the first complete account was propounded by Charles Darwin.
- Biogeographic distribution: Related species arise as geographic neighbors; this is the view that no new species arises except in close contact with its most related species. This view was proposed by Alfred Wallace and Charles Darwin. Of course, the fact that new species arise as biogeographic neighbors is explained by common ancestry, but Wallace formulated this model before the common ancestry model was published.
- The struggle for existence: More individuals will be born than the environment can support, so not all organisms survive to reproduce. Darwin derived this idea from Lyell, Thomas Malthus, and Alphonse de Candolle.
- Variation among individuals: All organisms generate offspring that are slightly different from each other, so there is variety within all populations. This is one of Darwin's original contributions to biology, although he was influenced by de Candolle.
- Natural selection: The local environment is more favorable to organisms with a particular variety or combination of traits within a species. Those so favored survive longer and reproduce more, resulting in that variety's becoming more common in subsequent generations than other varieties in the species. Darwin was not the first to recognize natural selection, but he was the first to use it as a mechanism of evolution.
- Sexual selection: In sexually reproducing species, mate choice sustains display traits — the sex that needs to compete for mates will show variations in the characteristics associated with this competition. Darwin is the sole author of this mechanism of evolutionary change.
- Random mutation, or blind variation: Changes arise in genes at random, without respect to the survival needs of organisms and species.
- Weismannism: Information from the somatic cells of the body is not inherited. This principle was proposed by August Weismann in 1882. The molecular genetic equivalent, due to Crick and Watson, is the Central Dogma of genetics: information is not passed from proteins to nucleotides (DNA or RNA).
- Drift: Many changes occur that are not selected for, due to sampling accidents in population. Models of drift were sketched in the 19th century by Darwin, George Romanes, and Moritz Wagner, but they came into their own with the advent of the Synthesis. There are different (and not necessarily incompatible) models of drift. One is genetic drift (after Sewall Wright). This includes genetic neutralism, which involves selectively neutral mutations becoming fixed in a population (after Mootoo Kimura). This includes "founder effect" models of speciation, formulated by Mayr and Hampton Carson.
- Canalization: Developmental processes are robust and resist change Models of canalization were proposed by Conrad Waddington and Ivan Ivanovich Schmalhausen). This notion is also sometimes called the "developmental constraints" model of evolution (see Schlichting and Pigliucci 1998).
- Mendelian genetics: Heredity is the passing on of discrete units of genetic material, which recombine in certain ways and frequencies, and which are either dominant or recessive. As the name implies, Mendelian genetics derive from the work of Gregor Mendel, as "rediscovered" by Correns, De Vries, and Tschermak in 1900 (Stern and Sherwood 1966). Mendelian genetics was incorporated into the synthesis by Fisher and Haldane.
- Rate variability: Evolution occurs at many different rates, from the instantaneous to the gradual, all of which are gradual and continuous at some scale. For example, GG Simpson (1944), Mayr (1942), and more recently, Niles Eldredge and Stephen Jay Gould (1972) in their famous punctuated equilibria model, examine how the pace of evolutionary change can vary under different circumstances.
- Process structuralism: Some changes are biased by their structural relations and form. The foundation of process structuralism was laid by D'Arcy Thompson (1917) and recently revived by Brian Goodwin (1994), and others. Complex structures and systems are not free to vary independent of their relationships with other components of the complex.
The long inferential chain
It is very hard to work out the implications of the effects of processes of local variation on large-scale ones. If gene frequency changes in local populations are the foundation for all evolution, it still may not be evident that a new species will arise, that there will be some trends in evolution, or that the patterns of life at any one time will reflect a process of speciation and retention of novelties in large groups.In part, this is because the chain of inference from population-level genetics to macroevolution is a very long one. Even if genes determined everything about species, we would not be able to generalize and elaborate these facts with any clarity or detail. We are limited in our ability to take all these things into account and lack the time and skills to work it all out fully. Reconciling the conservative character of genetic transmission with the tremendous potential for evolutionary change that is inherent in the genetic variation among individuals is particularly hard for those who lack a full appreciation of the way genes recombine and affect the development of organisms. This is especially true of secondary and post-secondary students and the general populace.
The allele-frequency definition, if adequate, would leave us unsatisfied that evolution really had been explained. Geneticists have observed in small scale a general resistance of the molecular components of the genome to change from the "norm" or "wild type". For this reason, if any biologist were to be anti-evolutionist, it would typically be one who works at the molecular level, such as a molecular geneticist or biochemist.
Allele frequencies change due to environmental factors acting on the population, to the relationships of mating within and between populations, and to sheer chance effects. To be able to predict accurately what will happen to a population in the future, one would need to have a full list of all the external influences on the population, along with knowledge of the size and structure of the population itself, including the mating behavior of the organisms within the population. Moreover, as Gould has emphasized (Gould 1989, 1996), contingency plays a large part in evolution, so that even if we had all this information, we could at best provide probabilities that specific outcomes might occur. The important thing to recognize is that we must consider the phenomenon of evolution simultaneously at many levels — the ecological, climatological, geological and developmental, as well as genetic.
Progress, or a lack of it
Since Lamarck, the view that evolution is progressive has been a recurring theme in biological writing. Even before Darwin published the Origin, his friend Herbert Spencer wrote that there was a general law of the "homogenous to the heterogeneous" — that is, life and the universe in general inevitably became more complex. Although some think differently (Ruse 1996), I believe that Darwin came to a contrary conclusion: that evolution had no direction or inevitability. Sometimes it seems that directionality does occur, but often this pattern is due to our own predilection for noticing only the emergence of large, obvious taxa, as when we refer to the "Age of the Reptiles" or "Age of the Mammals".Many people believe that evolution necessarily leads to complexity or specialized forms of life. Most organisms, however, are single-celled, and yet their adaptations to specific environments and predation are anything but "simple". Multicellular life is not the goal of evolution: indeed, it is a statistical blip in the overall diversity of living things. Of course, any increase over nothing is an increase, so it is obvious there has been some innovation in the course of evolution on any scale one cares to measure, but whether it progresses is the subject of massive debate (Nitecki 1988).
One form of progress that is generally accepted by most commentators is the "arms race" or "escalation" form of evolution, where two or more species coevolve as they attempt to get the edge over their predators or prey (Vermeij 1987). This is also called the "Red Queen" effect, from the character by that name in Through the Looking Glass who has to run as hard as she can just to stay in one place (Van Valen 1976).
However, the common misunderstanding is that there is a "next step" in evolution (usually, human evolution). This is our old friend the developmental sense of evolution, the scala naturae. Although popular with science fiction screenwriters, it has no scientific basis. It may be that if there is a next species that evolves from humans, it will be a herbivorous and apelike animal that survives just fine in post-apocalyptic conditions. Brains, for example, take a lot of energy to maintain. Brains bigger than ours may turn out to be a liability, not an asset.
WHAT EVOLUTION INCLUDES
To summarize, we can see that the concepts expressed by the word "evolution" have themselves evolved. There is change, there is phylogeny (the multiplication and extinction of lineages), there is selection and drift (the dynamics of reproducing populations), and there is the genetic and biological basis that underlies all these changes — all these (and more) have fallen under the rubric "evolution". Eli Minkoff (1983: 575) consolidated the contemporary understanding this way:Evolution
- Originally, a synonym for ontogeny....
- According to Lamarck and his contemporaries, the unfolding of (evolutionary) potentials as each species ascends the scala naturae.
- From 1809 on, the transformation of one species into another; phyletic evolution.
- According to many geneticists..., changes in the gene frequencies of populations.
- Anagenesis plus cladogenesis. Phylogeny and the changes in gene frequencies that produce phylogenetic change.