Why teach evolution? Here is one reason, from the introduction to the textbook, Evolution, that I coauthored:
Because closely related organisms tend to have similar biological features, evolutionary classification can help predict the biology of an organism through comparison with its relatives. The great majority of microbes on this planet have yet to be cultured, which makes it difficult to study their physiology. Using molecular biology methods, genes from unculturable species can be cloned and evolutionary analysis of those genes provides the information to place the unculturable microbes into a tree of life. This permits closely related species to be identified, including ones whose biology has been studied. For example, this approach has led to the development of new drugs to treat malaria.
The causative agent of malaria is a quite unusual organism in terms of its biology and also its evolutionary history, and understanding its unusual evolutionary history turns out to be critical for developing drugs to treat malaria.
Malaria is caused by the infectious eukaryotic parasite Plasmodium falciparum. Because humans are also eukaryotes, humans and P. falciparum share many aspects of their biology. Therefore drugs that have been developed to kill the parasite frequently are quite toxic to humans as well.
So the first part of this story is that, like us, Plasmodium falciparum is a eukaryote, a member of one of the major lineages of life on this planet. And this helps to explain why some drugs that target the malaria parasite are also toxic to humans.
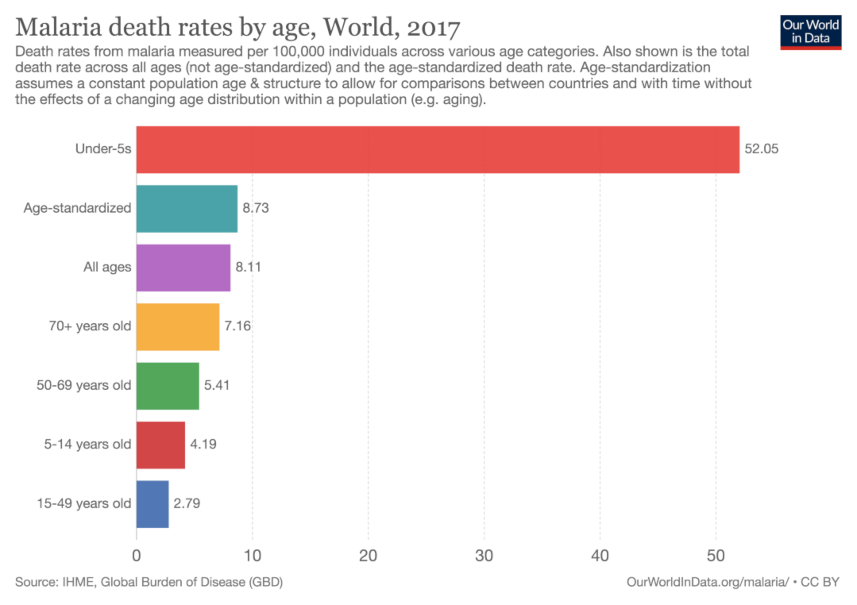